Acid rain: Difference between revisions
imported>Milton Beychok m (→Precipitation chemistry: Changed "chemistry" to "mechanism".) |
imported>Milton Beychok m (Added a reference moved from "Further Reading") |
||
Line 51: | Line 51: | ||
=== Acidity of the lakes and streams in the United States === | === Acidity of the lakes and streams in the United States === | ||
The National Surface Water Survey (NSWS) in the United States documented the status and extent of chronic acidification during surveys conducted from 1984 through 1988 in acid-sensitive regions throughout the United States. Many lakes and streams examined in the NSWS suffer from chronic acidity, a condition in which water has a constant low pH level. The survey investigated the effects of acidic deposition in over 1,000 lakes larger than 4 hectares (about 10 acres) and in thousands of miles of streams believed to be sensitive to acidification. Of the lakes and streams surveyed, acid rain caused acidity in 75 percent of the acidic lakes and about 50 percent of the acidic streams. Several regions in the United States were identified as containing many of the surface waters sensitive to acidification. They include the [[Adirondacks]] and [[Catskills]] in [[New York]] state, the mid-[[Appalachian]] highlands along the east coast, the upper midwest, and mountainous areas of the western United States. In areas like the northeastern United States, where the soil-buffering capacity is poor, some lakes now have a pH value of less than 5.<ref name=NSWS>[http://www.epa.gov/acidrain/effects/surface_water.html Effects of Acid Rain - Surface Waters and Aquatic Animals] The NSWS included an Eastern Lake Survey (published in 1986), a Western Lake Survey (published in 1987) and a National Stream Survey (published in 1988). The various reports published by the NSWS are available from the [[U.S. Environmental Protection Agency]].</ref> | The National Surface Water Survey (NSWS) in the United States documented the status and extent of chronic acidification during surveys conducted from 1984 through 1988 in acid-sensitive regions throughout the United States. Many lakes and streams examined in the NSWS suffer from chronic acidity, a condition in which water has a constant low pH level. The survey investigated the effects of acidic deposition in over 1,000 lakes larger than 4 hectares (about 10 acres) and in thousands of miles of streams believed to be sensitive to acidification. Of the lakes and streams surveyed, acid rain caused acidity in 75 percent of the acidic lakes and about 50 percent of the acidic streams. Several regions in the United States were identified as containing many of the surface waters sensitive to acidification. They include the [[Adirondacks]] and [[Catskills]] in [[New York]] state, the mid-[[Appalachian]] highlands along the east coast, the upper midwest, and mountainous areas of the western United States. In areas like the northeastern United States, where the soil-buffering capacity is poor, some lakes now have a pH value of less than 5.<ref name=NSWS>[http://www.epa.gov/acidrain/effects/surface_water.html Effects of Acid Rain - Surface Waters and Aquatic Animals] The NSWS included an Eastern Lake Survey (published in 1986), a Western Lake Survey (published in 1987) and a National Stream Survey (published in 1988). The various reports published by the NSWS are available from the [[U.S. Environmental Protection Agency]].</ref><ref>[http://www.maine.gov/dep/air/faqs.htm Frequently Asked questions] ''What is acid rain?'' ... and other acid rain questions, [[Maine]] Department of Environmental Protection, Bureau of Air Quality.</ref> | ||
Stream data from the HBEF reveal a number of long-term trends consistent with trends in lakes and streams across the northeastern United States.. Specifically, the concentration of sulfate in streams at the HBEF declined 20 percent between 1963-1994. The pH of streams subsequently increased from 4.8 to 5.0. Although this represents a significant improvement in water quality, streams at the HBEF remain acidic compared to background conditions, estimated to be above 6.0. Moreover, the ANC of the lakes and streams in the HBEF has not improved significantly over the past thirty years. | Stream data from the HBEF reveal a number of long-term trends consistent with trends in lakes and streams across the northeastern United States.. Specifically, the concentration of sulfate in streams at the HBEF declined 20 percent between 1963-1994. The pH of streams subsequently increased from 4.8 to 5.0. Although this represents a significant improvement in water quality, streams at the HBEF remain acidic compared to background conditions, estimated to be above 6.0. Moreover, the ANC of the lakes and streams in the HBEF has not improved significantly over the past thirty years. | ||
Line 137: | Line 137: | ||
* G.E. Likens, C. T. Driscoll, and D. C. Buso (1996). "Long-term effects of acid rain: Response and recovery of a forest ecosystem", ''Science'' 272:244-246. | * G.E. Likens, C. T. Driscoll, and D. C. Buso (1996). "Long-term effects of acid rain: Response and recovery of a forest ecosystem", ''Science'' 272:244-246. | ||
* ''NAPAP Biennial Report to Congress: An Integrated Assessment'', 1998, National Acid Precipitation Assessment Program, Silver Spring, Maryland. Available online [http://www.esrl.noaa.gov/csd/aqrs/reports/napapreport05.pdf here] on the website of the [[National Oceanic and Atmospheric Administration]]. | * ''NAPAP Biennial Report to Congress: An Integrated Assessment'', 1998, National Acid Precipitation Assessment Program, Silver Spring, Maryland. Available online [http://www.esrl.noaa.gov/csd/aqrs/reports/napapreport05.pdf here] on the website of the [[National Oceanic and Atmospheric Administration]]. | ||
== References == | == References == | ||
{{reflist}} | {{reflist}} |
Revision as of 18:36, 1 November 2010

Acid rain is a popular term for the atmospheric deposition of acidified rain, snow, sleet, hail and particulates, as well as acidified fog and cloud water. The increased acidity of these depositions, primarily from sulfuric and nitric acids, is generated as a by-product of the combustion of fuels,[1] especially in fossil fuel power plants. The heating of homes, electricity production, and driving vehicles all rely primarily on fossil fuel energy. When fossil fuels are combusted, acid-forming nitrogen oxides and sulfur oxides are released to the atmosphere. These chemical compounds are transformed in the atmosphere, often traveling thousands of kilometers from their original source, and then fall out on land and water surfaces as acid rain. As a result, air pollutants from power plants in the states of New Jersey or Michigan can impact pristine forests or lakes in undeveloped parts of the states of New Hampshire or Maine.[2]
Acid rain in North America was discovered in 1963 in rain at the Hubbard Brook Experimental Forest (HBEF)[3] that was some 100 times more acidic than unpolluted rain. Innovations for reducing fossil fuel combustion emissions, such as scrubbers upstream of the tall flue gas stacks on power plants and other industrial facilities, catalytic converters on automobiles, and use of low-sulfur coal, have been employed to reduce emissions of sulfur dioxide (SO2) and nitrogen oxides (NOx). As a result of the increasing global economies, fossil fuel combustion is increasing around the world, with concomitant spread of acid rain.
Terminology
The following definitions describe the technical terms that have a special meaning to the study or discussion of acid rain:[2][4]
Acid rain: A broad term that includes both wet and dry deposition of material from the atmosphere which contain higher than normal amounts of acids (predominantly sulfuric acid and nitric acid).
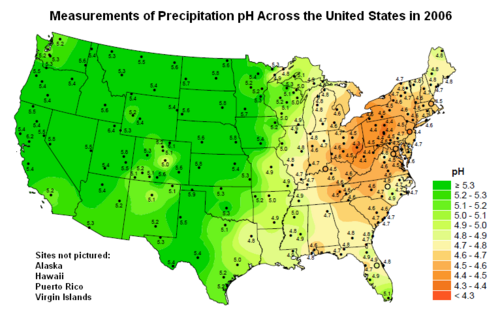
Map showing the pH of precipitation (wet deposition) across the United States.[5]
Wet deposition: A term that refers to acidic rain, snow, fog, or mist that falls onto the ground from the atmosphere.
Dry deposition: A term that refers to the acids that may become incorporated into the dust and other particulates in the atmosphere and fall onto the ground, buildings, homes, cars, and trees. Dry deposition is washed from these surfaces by rainstorms, causing an increase in the acidity of the runoff water. Dry deposition is difficult to quantify but has been estimated to represent 20% to 80% of the total acid deposition, depending on location, season, and total rainfall.[2]
Precipitation: A term that refers to either or both wet and dry deposition.
Acids: A term that refers to the acids found in acid rain. The atmosphere contains acid precursors (chemical forerunners) such as gaseous sulfur dioxide and nitrogen oxides that originate from natural sources as well as man-made (anthropogenic) sources. Those gases react with water and oxygen in the atmosphere to form mild (low concentration) solutions of sulfuric and nitric acids.
Acidity: The acidity of acid rain is measured using a scale called "pH". The lower is the pH of a substance, the higher is its acidity (i.e., the more acidic it is). The pH scale ranges from 0 (strongly acidic) to 14 (strongly alkaline or basic). Pure water has a pH of 7.0 which is considered to be "neutral", meaning that it is neither acidic or basic. However, normal rainfall is slightly acidic because carbon dioxide (CO2) in the atmosphere dissolves in the rain to form carbonic acid (H2CO3) which is weakly acidic and results in the rainfall having a pH of about 5.6.
Precipitation mechanism
- See also: Air pollution emissions
The combustion of fossil fuels in power plants and other industrial plants produces combustion flue gases, containing acid-forming precursors such as sulfur dioxide and nitrogen oxides, that are emitted to the atmosphere.. The combustion of hydrocarbon fuels in in the engines of automotive vehicles, aircraft, trains and ships also produce exhaust emissions of acid-forming precursors such as nitrogen oxides and, in some cases, sulfur dioxide. In addition to those anthropogenic (man-made) sources, natural sources such as trees, forest fires, volcanoes, and geysers also emit acid-forming nitrogen oxides.
Those acid-forming precursors, sulfur dioxide and nitrogen oxides, are converted in the atmosphere by a complex series of reactions, into sulfuric acid and nitric acid.[6]
The adjacent diagram depicts the emission of acid-forming precursors into the atmosphere and their conversion into sulfuric and nitric acid as well as their subsequent wet and dry deposition.
The emission of acid-forming precursors by natural sources led to the inference that precipitation in the pre-industrial atmosphere of forested regions had a pH of about 5.0 due only to the formation of carbonic acid from the CO2 in the atmosphere as per this reaction:
- water + carbon dioxide → carbonic acid
If that inference is true, then modern precipitation in the northeastern United States, ranging from about 4.5 to about 4.7 (see the above pH map), is two to three times more acidic than in pre-industrial times.
Effects of acid rain on surface water acidity
Acid deposition degrades the quality of surface water by increasing the acidity (i.e., lowering the pH) of the surface water. The degree of surface water acidification is an indicator of the potential harmful effects of acid rain on the biotic ecology as well as the plant life and soil of an entire watershed area.
Surface waters become acidic when the supply of acids from atmospheric deposition and other watershed processes exceeds the capacity of watershed soils and non-acidic drainage waters to neutralize them. When acidic surface waters drain into lakes and streams, it decreases their acid-neutralizing capacity (ANC).[7] Surface waters are technically defined as being ‘acidic’ if their acid neutralizing capacity is less than 0, which corresponds to having pH values less than about 5.2.
Aluminum leaches from silicate minerals which come in contact with low-pH waters and, hence, acidic surface waters increase the aluminum content of lakes and streams. While much of the aluminum present in surface waters is organically-bound and relatively non-toxic, certain inorganic species are highly toxic.
Acidity of the lakes and streams in the United States
The National Surface Water Survey (NSWS) in the United States documented the status and extent of chronic acidification during surveys conducted from 1984 through 1988 in acid-sensitive regions throughout the United States. Many lakes and streams examined in the NSWS suffer from chronic acidity, a condition in which water has a constant low pH level. The survey investigated the effects of acidic deposition in over 1,000 lakes larger than 4 hectares (about 10 acres) and in thousands of miles of streams believed to be sensitive to acidification. Of the lakes and streams surveyed, acid rain caused acidity in 75 percent of the acidic lakes and about 50 percent of the acidic streams. Several regions in the United States were identified as containing many of the surface waters sensitive to acidification. They include the Adirondacks and Catskills in New York state, the mid-Appalachian highlands along the east coast, the upper midwest, and mountainous areas of the western United States. In areas like the northeastern United States, where the soil-buffering capacity is poor, some lakes now have a pH value of less than 5.[8][9]
Stream data from the HBEF reveal a number of long-term trends consistent with trends in lakes and streams across the northeastern United States.. Specifically, the concentration of sulfate in streams at the HBEF declined 20 percent between 1963-1994. The pH of streams subsequently increased from 4.8 to 5.0. Although this represents a significant improvement in water quality, streams at the HBEF remain acidic compared to background conditions, estimated to be above 6.0. Moreover, the ANC of the lakes and streams in the HBEF has not improved significantly over the past thirty years.
Biologically-relevant surface water chemistry
The main cause for concern over the effects of surface water acidification in the United States. and elsewhere is the potential for detrimental biological affects. Typically, there is concern for biological impact if the pH is less than 6. At low pH values, aluminum may be present at concentrations that are toxic to biota, including sensitive life stages of fish and sensitive invertebrates. Aluminum is an abundant and normally harmless component of rocks and soils. However, it leaches from silicate minerals when they come in contact with low-pH waters. While much of the aluminum present in surface waters is organically-bound and relatively non-toxic, certain inorganic species are highly toxic. The best indicator of recovery in biologically-relevant chemistry would be a decrease in concentrations of inorganic monomeric aluminum, the most toxic form. Decreases in total aluminum would also suggest recovery, although the actual magnitude of the improvement in chemical conditions for biota would be unknown because we would not know how much of the decrease is due to inorganic vs. organic forms of aluminum.
Biological effects of acid rain
Effects on aquatic organisms
The biological effects of acidification have been demonstrated in laboratory and field bioassays, with whole-ecosystem acidification experiments, and through field surveys. A number of the species, especially of fish and macro-invertebrates, that commonly occur in surface waters susceptible to acidic deposition cannot survive, reproduce or compete in acidic waters. Sensitive species may be lost even at moderate levels of acidity. For example, some important zooplankton predators are not found at pH levels below 5.6; sensitive mayfly species (e.g., Baetis lapponicus) are affected at pH levels near 6.0; and sensitive fish species, such as the fathead minnow, experience recruitment failure and extinction at pH 5.6 to 5.9.
Decreases in pH and elevated concentrations of aluminum have reduced the species diversity and abundance of aquatic life in many streams and lakes in acid-sensitive areas of the northeastern United States. Fish have received most of the attention to date, but entire food webs are often adversely affected.
Decreases in pH and increases in aluminum concentrations have diminished the species diversity and abundance of plankton, invertebrates, and fish in acid-impacted surface waters in the northeastern United States.. In the Adirondacks, a significant positive relationship exists between the pH and acid-neutralizing capacity (ANC) of lakes and the number of fish species present in those lakes. Surveys of 1,469 Adirondack lakes conducted in 1984 and 1987 show that 24 percent of lakes (i.e., 346) in this region do not support fish. These lakes had consistently lower pH and ANC, and higher concentrations of aluminum than lakes that contained one or more species of fish. Even acid-tolerant fish species such as brook trout have been eliminated from some waters in the northeastern United States.
Acid episodes are particularly harmful to aquatic life because abrupt changes in water chemistry allow fish few areas of refuge. High concentrations of aluminum are directly toxic to fish and are a primary cause of fish mortality during acid episodes. High acidity and aluminum levels disrupt the salt and water balance in fish, causing red blood cells to rupture and blood viscosity to increase. Studies show that the viscous blood strains the fish’s heart, resulting in a lethal heart attack.
The absence of fish and the presence of aluminum in lakes provide important information about the condition of soils within a watershed. The release of aluminum from the soil into rivers and streams usually indicates that the available calcium in the soil is low and has been depleted. Furthermore, trees growing in such soils may experience greater nutritional stress.
Effect on soils
Acid deposition has altered and continues to alter soils in parts of the northeastern United States in three ways. Acid deposition depletes calcium and other base cations from the soil; facilitates the mobilization of dissolved inorganic aluminum (hereafter referred to simply as aluminum) into soil water; and increases the accumulation of sulfur and nitrogen in the soil.
Loss of calcium and other base cations
In the past 50-60 years, acid deposition accelerated the loss of large amounts of available calcium from soils in the northeastern United States. This conclusion is based on a limited number of soil studies, but at present, calcium depletion has been documented at more than a dozen study sites throughout the northeast, including sites in the Adirondacks, the White Mountains, the Green Mountains, and the state of Maine. Depletion occurs when base cations are displaced from the soil by acid deposition at a rate faster than they can be replenished by the slow breakdown of rocks or the deposition of base cations from the atmosphere. This depletion of base cations fundamentally alters soil processes, compromises the nutrition of some trees, and hinders the capacity for sensitive soils to recover.
Mobilization of aluminum
Aluminum is often released from soil to soil water, vegetation, lakes, and streams in forested regions with high acid deposition, low stores of available calcium, and high soil acidity. High concentrations of aluminum can be toxic to plants, fish, and other organisms. Concentrations of aluminum in streams at the Hubbard Brook Experimental Forest, New Hampshire (HBEF) are often above levels considered toxic to fish and much greater than concentrations observed in forested watersheds receiving low levels of acid deposition.
Accumulation of sulfur and nitrogen
Acid deposition results in the accumulation of sulfur and nitrogen in forest soils. As sulfate is released from the soil, it acidifies nearby streams and lakes. The recovery of surface waters in response to emission controls has therefore been delayed and will not be complete until the sulfate left by a long legacy of acid deposition is released from the soil.
Similarly, nitrogen has accumulated in soil beyond the amount needed by the forest and appears now to be leaching into surface waters in many parts of the northeastern United States. This process also acidifies lakes and streams. Forests typically require more nitrogen for growth than is available in the soil. However, several recent studies suggest that in some areas, nitrogen levels are above what forests can use and retain.
Effects on forest ecosystems
The 1990 National Acid Precipitation Assessment Program (NAPAP) report to Congress concluded there was insubstantial evidence that acid deposition had caused the decline of trees other than red spruce growing at high elevations. More recent research shows that acid deposition has contributed to the decline of red spruce trees throughout the eastern United States and sugar maple trees in central and western Pennsylvania. Symptoms of tree decline include poor crown condition, reduced tree growth, and unusually high levels of tree mortality. Red spruce and sugar maple are the species that have been most intensively studied.
Red Spruce
Since the 1960s, more than half of large canopy red spruce in the Adirondack Mountains of New York and the Green Mountains of Vermont and approximately one quarter of large canopy red spruce in the White Mountains of New Hampshire have died. Significant growth declines and winter injury to red spruce have been observed throughout its range. Acid deposition is the major cause of red spruce decline at high elevations in the northeas ern United States. Red spruce decline occurs by both direct and indirect effects of acid deposition. Direct effects include the leaching of calcium from a tree’s leaves and needles (i.e., foliage), whereas indirect effects refer to changes in the underlying soil chemistry.
Recent research suggests that the decline of red spruce is linked to the leaching of calcium from cell membranes in spruce needles by acid rain, mist or fog. The loss of calcium renders the needles more susceptible to freezing damage, thereby reducing a tree’s tolerance to low temperatures and increasing the occurrence of winter injury and subsequent tree damage or death. In addition, elevated aluminum concentrations in the soil may limit the ability of red spruce to take up water and nutrients through its roots. Water and nutrient deficiencies can lower a tree’s tolerance to other environmental stresses and cause decline.
Sugar Maple
The decline of sugar maple has been studied in the eastern United States since the 1950s. Extensive mortality among sugar maples in Pennsylvania appears to have resulted from deficiencies of base cations, coupled with other stresses such as insect defoliation or drought. According to research studies, the probability of the loss of sugar maple crown vigor or the incidence of tree death increased on sites where supplies of calcium and magnesium in the soil and foliage were the lowest and stress from insect defoliation and/or drought was high. In northwestern and north central Pennsylvania, soils on the upper slopes of unglaciated sites contain low calcium and magnesium supplies as a result of more than half a million years of weathering combined with the leaching of these elements by acid deposition. Low levels of these base cations can cause a nutrient imbalance and reduce a tree’s ability to respond to stresses such as insect infestation and drought.
Trends in acidification
Regulatory controls initiated in the 1970s and 1990s have had an important impact on the emissions of pollutants that cause acid rain. Especially important is the Acid Rain Program in Title IV of the United States' Clean Air Act. The Acid Rain Program has the goals of lowering the electric power industry’s annual emissions of (1) sulfur dioxide (SO2) to half of 1980 levels, capping them at 8.95 million tons starting in 2010, and (2) nitrogen oxide (NOx) to 2 million tons lower than the forecasted level for 2000, reducing annual emissions to a level of 6.1 million tons in 2000. In fact, SO2 emissions decreased 33 percent from 1983 to 2002 and 31 percent from 1993 to 2002.
Over the past few decades:
- Ambient SO2 and sulfate levels are down more than 40 percent and 30 percent, respectively, in the eastern United States.
- Wet sulfate deposition, which acidifies sensitive lakes, streams and forest soils, has decreased 39 percent in the northeastern United States and 17 percent in the southeastern United States.
- Some modest reductions in inorganic nitrogen deposition and wet nitrate concentrations have occurred in the Northeast and Mid-Atlantic regions of the United States, but other areas have not shown much improvement.
These trends indicate significant progress, but acid rain remains a significant long-term issue in the United States and elsewhere. Importantly, the emission and atmospheric deposition of base cations that help counteract acid deposition have declined significantly since the early 1960s with the enactment of pollution controls on particulate matter, and the ability of ecosystems to neutralize acid deposition has decreased in some regions. Consequently, lakes, streams, and soils in many parts of the northeast are still acidic and exhibit signs of degradation linked to acid deposition.
Ecosystem recovery from acid deposition
Recovery from acid deposition involves decreases in emissions resulting from regulatory controls, which in turn lead to reductions in acid deposition and allow chemical recovery. Chemical recovery is characterized by decreased concentrations of sulfate, nitrate, and aluminum in soils and surface waters. If sufficient, these reductions will eventually lead to increased pH and acid-neutralizing capacity (ANC), as well as higher concentrations of base cations. As chemical conditions improve, the potential for the second phase of ecosystem recovery, biological recovery, is greatly enhanced.
An analysis of the scientific literature suggests that the following five thresholds can serve as indicators of chemical recovery. If chemical conditions in an ecosystem are above these thresholds, (or in the case of aluminum, are below the threshold) it is unlikely that the ecosystem has been substantially impaired by acid deposition. Conversely, if chemical conditions are below these thresholds, (or in the case of aluminum, above the threshold) it is likely that the ecosystem has been, or will be, impaired by acid deposition.
As chemical conditions in soils and surface waters improve, biological recovery is enhanced. Biological recovery is likely to occur in stages, since not all organisms can recover at the same rate and may vary in their sensitivity to acid deposition. The current understanding of species’ responses to improvements in chemical conditions is incomplete, but research suggests that stream macro-invertebrates may recover relatively rapidly (i.e., within 3 years), while lake zooplankton may need a decade or more to fully re-establish. Fish populations in streams and lakes should recover in 5-10 years following the recovery of the macro-invertebrates and zooplankton which serve as food sources. It is possible that, with improved chemical conditions and the return of other members of the aquatic food web, the stocking of streams and lakes could help to accelerate the recovery of fish.
Terrestrial recovery is even more difficult to project than aquatic recovery. Given the life span of trees and the delay in the response of soil to decreases in acid deposition, it is reasonable to suggest that decades will be required for affected trees on sensitive sites to recover once chemical conditions in the soil are restored.
The time required for chemical recovery varies widely among ecosystems in the northeastern United States, and is primarily a function of:
- the historic rate of sulfur and nitrogen deposition;
- the rate and magnitude of decreases in acid deposition;
- the extent to which base cations such as calcium have been depleted from the soil;
- the extent to which sulfur and nitrogen have accumulated in the soil and the rate at which they are released as deposition declines;
- the weathering rate of the soil and underlying rock and the associated supply of base cations to the ecosystem; and
- the rate of atmospheric deposition of base cations.
Further reading
- Hubbard Brook Ecosystem Study Homepage
- C. T. Driscoll et al (2001). Acid Rain Revisited: advances in scientific understanding since the passage of the 1970 and 1990 Clean Air Act Amendments. A publication of the Hubbard Brook Research Foundation.
- G.E. Likens, T. J. Butler, and D. C. Buso (2000). "Long- and short-term changes in sulfate deposition: effects of the 1990 Clean Air Act Amendments", Biogeochemistry, (52)1:1-11.
- G.E. Likens, F. H. Bormann, and N. M. Johnson (1972). "Acid rain", Environment 14:33-40.
- G.E. Likens, C. T. Driscoll, and D. C. Buso (1996). "Long-term effects of acid rain: Response and recovery of a forest ecosystem", Science 272:244-246.
- NAPAP Biennial Report to Congress: An Integrated Assessment, 1998, National Acid Precipitation Assessment Program, Silver Spring, Maryland. Available online here on the website of the National Oceanic and Atmospheric Administration.
References
- ↑ Note: Sulfuric acid is formed from the sulfur dioxide resulting from combustion of sulfur-containing fuels. Nitric acid is formed from the nitrogen oxides resulting from the high temperature partial conversion of the nitrogen contained in the combustion air.
- ↑ 2.0 2.1 2.2 The primary source for this article was Acid Rain August 7, 2010 (last revised October 19, 2010), Gene Likens (Lead author), Wayne Davis, Lori Zaikowski and Stephen C. Nodvin. (Published on the website of the Encyclopedia of Earth)
- ↑ Note: Site of the Hubbard Brook Ecosystem Study in the White Mountains of New Hampshire
- ↑ Acid rain From the website of the U.S. Environmental Protection Agency
- ↑ National Atmospheric Deposition Program 2006 Annual Summary
- ↑ Acid Deposition Bulletin American Meteorological Society, 85, 299—301, adopted by the American Meteorological Society, September 2003
- ↑ Note: The acid-neutralizing capacity (ANC) of a lake or stream is analogous to the alkalinity of the lake or stream
- ↑ Effects of Acid Rain - Surface Waters and Aquatic Animals The NSWS included an Eastern Lake Survey (published in 1986), a Western Lake Survey (published in 1987) and a National Stream Survey (published in 1988). The various reports published by the NSWS are available from the U.S. Environmental Protection Agency.
- ↑ Frequently Asked questions What is acid rain? ... and other acid rain questions, Maine Department of Environmental Protection, Bureau of Air Quality.
- Editable Main Articles with Citable Versions
- CZ Live
- Biology Workgroup
- Chemistry Workgroup
- Chemical Engineering Subgroup
- Environmental Engineering Subgroup
- Articles written in American English
- Advanced Articles written in American English
- All Content
- Biology Content
- Chemistry Content
- Chemical Engineering tag
- Environmental Engineering tag