Fossilization (palaeontology): Difference between revisions
imported>Meg Taylor No edit summary |
Pat Palmer (talk | contribs) |
||
Line 53: | Line 53: | ||
Fossils represent a very small proportion of all the organisms which have ever lived. This is because fossils only form under specific conditions. [[Decomposition]] is a requirement for environments to function, and so fossilization represents a deviation from this natural process.<ref name="MacRae"/> | Fossils represent a very small proportion of all the organisms which have ever lived. This is because fossils only form under specific conditions. [[Decomposition]] is a requirement for environments to function, and so fossilization represents a deviation from this natural process.<ref name="MacRae"/> | ||
Destructive agents which aid decomposition include predators and scavengers, transport by wind and water, weathering by changes in temperature, wind, rain and chemicals, and decay by microbes, [[ | Destructive agents which aid decomposition include predators and scavengers, transport by wind and water, weathering by changes in temperature, wind, rain and chemicals, and decay by microbes, [[insect]]s and roots.<ref name="Shipman">Shipman, P. 1981. ''Life History of a Fossil: An Introduction to Taphonomy and Paleoecology''. Harvard University Press, England.</ref> Various agents can be responsible for the preservation of organisms. Commonly, sediments bury the remains and keep them from being disturbed during the process of fossilization. More unusual agents include lava or volcanic ash, and severe cold or aridity.<ref name="Shipman"/> Temperatures below 4°C (39.2ºF) inhibit bacterial activity and thus, decay of organic matter.<ref name="Allison & Briggs"/> | ||
Generally, the following circumstances are favorable for fossilization: | Generally, the following circumstances are favorable for fossilization: |
Revision as of 09:30, 24 July 2024

Fossilization is the process that preserves evidence of life in earth's rock record. This evidence of past life is called a fossil. The word "fossil" is derived from the Latin fossilis, something dug up.[1] During the Middle Ages, the term "fossil" was used for any sample recovered from the earth, including rocks and minerals.[1] Today, the use of "fossil" is limited to the record of ancient life. Fossilization can preserve actual remnants of an organism, or evidence of their presence in an ecosystem.[1]
Understanding the process of fossilization, and the different means through which it can occur, is used by taphonomists, paleontologists, and geologists to understand the lives of past organisms and the ancient environments in which they lived. The fossil record, however, is not a representative sample of past life, because certain organisms, and particular elements of those organisms, are more likely to be preserved than others. In addition certain environments are more suitable for fossilization than others, and some environments will yield no fossil remains through time.
Taphonomy is the study of what has happened to an organism from the moment of death until it is found as a fossil.[2] Exploring the process of fossilization from a taphonomic perspective allows placement of individual fossils into a wider context. Consequently, researchers reconstruct not only the morphology of organisms, but under the right conditions, their behavior, life history and palaeoenvironment as well.
Overview
Organisms pass through a number of stages on their way to becoming fossils.[3]
Stage | Definition |
---|---|
Death | Generally, this is the first stage in fossilization. Death can occur as a result of burial and in this case biostratinomic processes may not take place. |
Biostratinomic processes | Processes such as reorientation, disarticulation, fragmentation, and corrosion, which modify the remains. Not desirable, as information is lost. |
Deposition | Burial of the remains in sediment, where they are less likely to be disturbed. |
Diagenetic processes | Chemical / physical changes e.g. permineralization. Necessary for fossilization. |
First, the organism must be deposited in sediment. Between the time of death and burial, biostratinomic processes alter the remains. Before burial, an organic skeleton is normally subject to reorientation, disarticulation, fragmentation, and corrosion. Once it is buried, it undergoes diagenetic processes. Diagenesis is simply any change, chemical or physical, which occurs in an organism after burial.[3] Such changes are necessary for preservation, because organic matter will not survive for long before it is decomposed, and even hard parts, as bones, teeth, calcified shells, are normally prone to destruction. A typical diagenetic process is mineralization, which can occur with various minerals such as pyrite, phosphates or the various forms of silica.[3]
Fossilization is not a process that only occurred millions of years ago. It has also occurred in the recent past, simply because the same geological processes that happened in the past are also taking place now. This is called the Principle of Uniformitarianism.[4] For example, Anna Behrensmeyer reported bones in all stages of fossilization, from organic to completely mineralized, in the Amboseli Basin of East Africa.[5] Bones are dated from the Holocene to the Pleistocene, negating the claim made by some that no instances of fossilization are apparent today.
“Post-mortem modification is a virtually unavoidable fact of fossilization”.[6] Older fossils are more likely to have been modified by diagenetic processes, and thus, to be a less accurate copy of the original.[6] Analysis of modes of fossilization in an assemblage can reveal whether that assemblage represents input from more than one source.[7]
Fossilization of organic tissues
There are five general types of fossilization:[6]
- carbonized soft tissue
- diagenetically mineralized three-dimensional tissue
- tissue outlines
- carbonized refractory tissue
- originally mineralized tissue, i.e., shell and bone.
Carbonization is a process occurring within the sediment in the absence of oxygen. In such conditions, anaerobic bacteria consume hydrogen and oxygen, therefore concentrating carbon. As long as the sediment or rock is kept in anoxic environments, the carbon, which is residual organic tissue, is preserved, but carbonized soft tissue is only preserved as a thin film. The final products of carbonization are coal and hydrocarbons, which can be considered accumulations of very badly preserved fossils.
Permineralization and petrification are processes leading to the preservation of tissues in three dimensions. They are common, for example, in bones which have a high porosity and are filled with marrow. If bones become buried when an animal dies, ground water can permeate. Carbonate minerals such as calcite, or silica, precipitate out of solution and solidify the organic material, accurately preserving the internal cellular structure. This is the process of permineralization, and has occurred in most older fossils.[1] In permineralization, the cell pores are packed with minerals, while the walls remain highly organic.[6] Replacement happens when minerals replace the organic matter completely. The mineral replacement of shell or bone is also called petrification, because it turns organic material into stone.[4] When a fossil is petrified, both the cell walls and voids are mineralized.[6] Another example of petrification is silicified wood. Around 20 different minerals have been found to replace fossils. Chalcedony, pyrite and iron oxide are common in replacement.[1]
An organism can leave a mold behind when it decays, preserving surface details in the surrounding sediment. If the mold later fills with sediment, a replica of the organism is created, called a natural cast. A natural cast does not preserve the internal or cellular structure of the original organism, but only its surface detail.[1] The term cast is also used to indicate three-dimensional copies of a fossil, made out of plaster and resin. These casts are useful for teaching purposes, or when the original fossil is fragile or damaged. However, these laboratory casts are created in a similar way to a natural cast, by using a mold. The endocranial cast of the Taung child was formed naturally by the accumulation of lime inside the skull, and preseves the sutures of the brain on its surface.
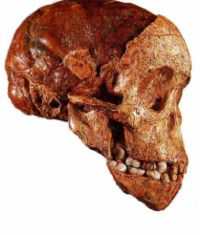
Partial skull and endocranial cast of the Taung Child, holotype of Australopithecus africanus.
Carbonized refractory tissue is, e.g., charcoal.
Finally, some hard tissues, as bone or shell, may resist diagenesis and preserve substantially unmodified. This is relatively common for Quaternary fossils, but becomes an exceptionally rare instance for Tertiary or older fossils.
Trace fossils are not remnants of an organism itself, but some record of its behaviour. Tracks, burrows, coprolites (fossil feces), and stone tools are all examples of trace fossils. The study of fossil traces is a special branch of paleontology, called ichnology.
Conditions for preservation
Fossils represent a very small proportion of all the organisms which have ever lived. This is because fossils only form under specific conditions. Decomposition is a requirement for environments to function, and so fossilization represents a deviation from this natural process.[1]
Destructive agents which aid decomposition include predators and scavengers, transport by wind and water, weathering by changes in temperature, wind, rain and chemicals, and decay by microbes, insects and roots.[2] Various agents can be responsible for the preservation of organisms. Commonly, sediments bury the remains and keep them from being disturbed during the process of fossilization. More unusual agents include lava or volcanic ash, and severe cold or aridity.[2] Temperatures below 4°C (39.2ºF) inhibit bacterial activity and thus, decay of organic matter.[6]
Generally, the following circumstances are favorable for fossilization:
- The organism should be rapidly buried to avoid damage by scavengers or weathering agents like water, wind and fluctuations in temperatures.[4] Thus, fossilization is much more common in marine environments, which are characterized preferentially by sediment deposition rather than erosion. In continental environments, fossils are most common in fluvial sediments, and rare in soils.[2] The remains require little or no disturbance during the fossilization process.
- The organism should be buried in anaerobic conditions, because oxygen encourages the presence of bacteria which decompose organic material.[4] Examples of continental anaerobic environments include wet areas like swamps and floodplains. However, decay can occur under anoxic conditions, for example by bacterial sulfate reduction.[8] Anoxia also creates reducing conditions, forming reactive ionic species. This encourages mineral precipitation.[8]
- Containing some hard parts increases the likelihood that an organism will be preserved, but it is not necessary for fossilization.[9] Although multicellular life was already diversifying well before the Cambrian period, an extensive record of pre-Cambrian life forms does not exist.[4] It was only during the Cambrian Explosion of life that many forms began simultaneously covering themselves with a hard exterior. This hard surface developed as protection against predation, but also happened to be conducive to fossilization.[4]
Because of the specific conditions needed for a fossil to be created, organisms that do not live in environments favorable to preservation are likely never to appear in the fossil record. Small, gracile animals are normally too delicate to withstand the ravages of time, as are animals living in a high-acidic environment like a tropical forest.[10] Most depositional environments are marine, while terrestrial environments tend to be erosional. Thus, a much better fossil record exists for marine organisms than for terrestrial life.[1]
Diagenetic processes
Silification
Silica, and especially its most common crystallized form (the mineral quartz), is extremely stable at the Earth’s surface, therefore silicified fossils are likely to withstand modification or damage.[6] Silification occurs mainly in plants, while in soft organisms it is very rare.[8] When plant matter is silicified, this is an example of permineralization and not replacement.[11] There are four variations on silification replacement: mosaic quartz, spherulitic chalcedony, lutecite and microgranular quartz.[6] Leo & Barghoon proposed that partial decay is necessary for silification, although it may be only on the molecular level.[11]
Phosphatization
Phosphatization [8] of soft parts have an unsurpassed degree of preservation, with detailed cellular structure. Muscle fibers are most often preserved through phosphate replacement. Normally carbonate ion concentrations are higher than phosphates in both fresh and marine waters, and so carbonate replacement is preferred. For phosphatization to occur, organic content of the water must be high, coupled with a low rate of burial.
Pyritization
Pyritization often happens in conjunction with permineralization and replacement, as does silification.[9] It requires an availability of dissolved iron.[6] Soft tissue can be preserved by pyrite in three ways: permineralization, mineral coating or pseudomorphs, and mineral casts or moulds.[8] Although both mineral coating and casts preserve only surface detail, that of coating is far more detailed. Pyritized soft tissue is very rare.[8]
Carbonate preservation
Carbonate preservation [6] is influenced by predation, water chemistry, shell morphology and mineralogy, and shell dissolution kinetics. Surrounding water must be saturated with carbonates in order to avoid dissolution of carbonate skeletal remains. Ideal conditions for carbonate preservation are normally found in marine shelf sediments, with a high biomass of organisms that could potentially fossilize. In fresh water, concentrations of calcium carbonate vary considerably, and so preservation in these environments is not as predictable. Carbonate preservation in freshwaters may be referred to as a transient state, because even minor changes in water chemistry, temperature, or pressure may be sufficient to destroy all preservation present at that time. Consequently, it may seem astonishing that carbonate preservation occurs at all. There are three mitigating factors: carbonate dissolution occurs much quicker than replacement by other minerals, fine-grained sediments will protect the process from ex situ fluctuations, and cementation of the sediment enhances preservation. On the contrary, normal marine waters are constantly supersaturated with respect to calcium carbonate, and the preservation of carbonate shells is a rather common instance.
Charcoalification
Charcoalification [6] can preserve tissues burned in wildfires or buried in pyroclastic flows. Internal structure is often detailed. Even though charcoal is soft, it is easily transported because of its lightness, which may explain its survival to present-day.
The fate of biomolecules during fossilization
The field of molecular palaeontology is new, but can provide important information about diagenetic processes, and allow us to identify stratigraphic biomarkers.[6] Molecules can also be diagnostic of certain taxonomic groups. Nucleic acids and proteins are the most subject to decay, while lipids, resin, lignin and cutan are highly resistant. However, polymerase chain reaction (PCR) has now allowed DNA and RNA to be recovered from some fossils, although it would never be stable enough to clone extinct organisms.[6]
Fossilization in different organisms
Because biomolecules are affected differently during the decay process, the makeup of organisms influences their potential to become fossilized. Organisms composed largely of unstable elements such as fat and muscle are much less likely to enter the fossil record then organisms with a substantial component of stable tissue like bone or shell.[1] Animals like jellyfish, composed entirely of soft tissue, rarely fossilize, but impressions, another kind of fossil, are evident in ocean floor sediments and confirm their existence. It is practical to group organisms according to how they fossilize. Soft-bodied invertebrates, shelled organisms, plants and vertebrates all have distinctive reactions to taphonomic processes.[6]
Soft tissue preservation
Because soft tissue is so nutritious for predators, scavengers and microbes, its fossilization is very rare compared to that of hard parts. Preservation of soft tissue can also influence that of skeletal elements, by keeping them articulated.[6] Rapid burial is not usually important in soft body fossilization.[8] Cyanobacteria and other microbia are instrumental in preserving soft organisms by means of microbial films.[12]
Plant fossilization
When plants are fossilized, they are normally not found intact. Various structures of a plant are inclined to fall off during its lifetime, creating separate fossils of the different parts.[4] Leaves and fruits are shed, and pollen can disperse far away from the original plant. Unless these structures are found together on the same plant, they are given different names, as it cannot be certain they are the same taxon.[4]
Most plants are preserved as compressions or impressions, and often coated with minerals like iron oxide.[6] Impression fossils are created when the groundwater is alkaline and oxygen is present. All the plant material is destroyed, but a highly detailed impression remains if the surrounding sediment was fine-grained.[1] Compression is a special case of carbonization. For a compression, ground water must be acidic, with no oxygen present, to ensure preservation. After burial, the soft and thin-walled tissue of leaves decays quickly, but the outer cuticle remains.[1] Successive layers of sediment cover plant material, increasing temperature and pressure. This drives off the unstable components of the remaining organic molecules, carbonising the material, which appears as a black film of coal.[1] Pollen and spores are very small and easily trapped in sediments.[4] They also require acidic, anoxic conditions for preservation, and have an outer protective layer of exine resistant to acid. Petrified wood forms when tree trunks were submerged in waters rich in minerals.[4] Over time, these minerals replaced the organic matter, maintaining the internal cellular structure as well as the external morphology. Silicification, pyritization and carbonate mineralization are common among plant fossils, particularly in wood.[6]
Hard tissue fossilization
Carbonate shells and tests of marine organisms as molluscs, brachiopods, echinoderms, foraminifers are, by far, the most common fossils, thanks to their resistance to taphonomic and diagenetic alteration. Nevertheless, weakly acidic water often dissolve a shell completely. If the sediment surrounding the shell has already been consolidated, the surface details of the shell will be imprinted on the rock, forming internal and external molds. The relief of the mold is opposite to that of the original shell.[1] When the sediment is partially unconsolidated when the shell dissolves, the sculptures of the external and internal sides overlap, and results in a composite mould. Shells often undergo recrystallization, where the composition remains unaltered, but the crystal structure is changed. Calcium carbonate crystals become coarser and larger, and the original microstructure is not preserved.[1] The most common vertebrate fossils are bones and teeth.[2] Both the structure of the skeletal element, and the taphonomic processes which it is subjected to, affect its chances of passing into the fossil record. This is termed the preservation potential.
Preservation potential
The concept of preservation potential can be explained with the example of vertebrate bones.
Preservation potential [2] is primarily determined by the bone itself, rather than by what happens to it. A bone’s size, shape, how it weathers, and breaks when chewed are all a result of its structural properties. Small bones, bones with a high marrow content, cancellous bone, and bones intimately associated with soft tissue (e.g. ribs) have the lowest preservation potential. This can create a biased taphonomic assemblage. For example, an assemblage consisting only of large-boned, savanna animals does not necessarily indicate a savanna environment. Smaller-boned forest animals are much less likely to be preserved, but they could have been present in life.
Taphonomic processes are also important in determining whether an animal will enter the fossil record. The cause of death, in particular, directly impacts upon an animal’s chance of fossilization. If an animal is predated upon, at least some of the carcass will be eaten, reducing its chance of being preserved. However, some predators are less destructive than others when it comes to feeding. The fact that some fossils have been found with tooth marks means that it is possible for a predated animal to enter the fossil record.[2] Disease as a cause of death has little impact on the likelihood of fossilization. Dying of old age may mean that the animal’s bones have lost minerals with age, but this could be offset that they have grown to a larger size. Larger bones are more likely to withstand destructive agents. Accidental deaths are frequently excellent for preservation. Many an animal has been trapped in tar pits, soft mud or volcanic lava, all of which ensure rapid burial. Falling into a cave would ensure the skeleton is kept together, and protect it from predation if the cave was inaccessible. In limestone caves, bones may be lithified into breccias, such as at the Cradle of Humankind.
If animals die of starvation or dehydration, a drought was likely to be the cause. During drought conditions, animals refuse to leave a water source even if no forage is left. The simultaneous death of numerous animals near a water source is extremely likely to pass into the fossil record. Firstly, when the drought ends, water levels will rise again and could bury the carcasses. Secondly, the surplus of food for predators means that not all the animals would be consumed.
Extraordinary instances of preservation
In unusual circumstances, more than just the hard parts of an organism may be preserved.
- Whole frozen mammoths have been retrieved from permafrost in Siberia, with skin and hair intact.[13] Permafrost has preserved the mammoths so well that their flesh is judged edible.[13]
- Arid climates can sometimes naturally mummify animals, preserving skin and bone, in the same manner as mummification practiced in Ancient Egypt.[4]
- Amber has been made famous for its preservation properties in the movie Jurassic Park. Amber forms when plant resin is exposed to the air, causing its constituent molecules (terpenes) to cross-link and harden.[9] The substance is very resistant to decay, with the oldest amber found dating to the Late Carboniferous (290 Ma). Amber younger than 40 000 years is termed “sub-fossil resin”.[14] Ambers from different localities can be recognised by their chemical make-up, and also distinguished by spectroscopy.[15] This is highly useful if the amber has plants or animals trapped inside. Organisms are 3-dimensional with excellent morphological preservation, but their internal structure is dissolved.[14]
- Peat bogs have yielded the famous “Bog People” – two thousand year old humans with intact skin, hair and clothing due to the presence of anti-bacterial tannic and fulvic acids.[8] Because of the low pH, their bones have been dissolved.[2]
There are some extraordinary instances of preservation called Lagerstätten, and can either be concentration deposits (mainly hard parts like shell and bone), or conservation deposits, which have preserved soft-bodied organisms.[9]
- The Grube Messel in Germany was a shallow but highly anoxic lake, deposited during the Middle Eocene.[16] Algal decay and plankton blooms were responsible for the anoxic conditions, resulting in excellent preservation of many birds and bats, which normally don’t fossilize well because of their fragile skeletons. Diatoms, pollen, insects, and all classes of vertebrates have also been found, most articulated.[16] Outlines of bodies as well as digestive contents are sometimes visible.
- The Rhynie Chert is an ancient hot spring in Scotland, dating to the Early Devonian. Rapid silification occurs in hot spring sites, so they are excellent for fossil finds.[17] The Rhynie hot spring is highly significant because the earliest terrestrial communities were forming during this time.[17] Flooding of the spring periodically captured the structure of the community, which included the plant Rhynia, algae, and various arthropods.
- The Middle Cambrian Burgess shale, in the Canadian rocky mountains, is perhaps the most known fossil lagerstätten. Here, a variety of marine organisms are preserved with mineralized soft tissue. The majority of them belong to extinct taxa.[18]
- The Upper Jurassic lagerstätten of Solnhofen, Germany, was a marginal marine setting with anoxic sea bottom. The absence of oxygen and fine sediments allowed the fine preservation of delicate soft body parts. Its position close to the coast line implies that marine and continental organisms are found associated at Solnhofen. The site is famous for the finding of Archaeopteryx, which is considered the oldest known bird. At least some of the specimens exhibit preserved feathers.
References
- ↑ 1.00 1.01 1.02 1.03 1.04 1.05 1.06 1.07 1.08 1.09 1.10 1.11 1.12 1.13 MacRae, C.S. 1999. Life Etched in Stone: Fossils of South Africa. The Geological Society of South Africa, Johannesburg.
- ↑ 2.0 2.1 2.2 2.3 2.4 2.5 2.6 2.7 Shipman, P. 1981. Life History of a Fossil: An Introduction to Taphonomy and Paleoecology. Harvard University Press, England.
- ↑ 3.0 3.1 3.2 Brett, C.E. & Baird, G.C. 1986. Comparative Taphonomy: A Key to Paleoenvironmental Interpretation Based on Fossil Preservation. PALAIOS 1(3): 207-227.
- ↑ 4.00 4.01 4.02 4.03 4.04 4.05 4.06 4.07 4.08 4.09 4.10 McCarthy, T. & Rubidge, B. 2005. The Story of Earth and Life: A southern African perspective on a 4.6-billion-year journey. Struik Publishers, Cape Town.
- ↑ Behrensmeyer, A.K. 1991. Vertebrate Paleoecology in a Recent East African Ecosystem. In: Gray, J., Boucot, A.J. & Berry, W.B.N. (eds.), Communities of the Past, Stroudsburg: Hutchinson Ross Publishing Co.
- ↑ 6.00 6.01 6.02 6.03 6.04 6.05 6.06 6.07 6.08 6.09 6.10 6.11 6.12 6.13 6.14 6.15 6.16 Allison, P.A. & Briggs, D.E.G. 1991. Taphonomy: Releasing the Data Locked in the Fossil Record. Plenum Press, New York.
- ↑ Henderson, P., Pickford, M. & Williams, C.T. 1987. A geochemical study of rocks and spring waters at Kanam and Kanjera, Kenya, and the implications concerning element mobility and uptake. Journal of African Earth Sciences 6: 221-227.
- ↑ 8.0 8.1 8.2 8.3 8.4 8.5 8.6 8.7 Allison, P.A. 1988. Konservat-Lagerstätten: cause and classification. Paleobiology 14: 331-334.
- ↑ 9.0 9.1 9.2 9.3 Martin, R.E. 1999. Taphonomy: A Process Approach. Cambridge University Press.
- ↑ DiSilvestro, D.L. 1997. In Quest of the Origin of Birds. BioScience 47(8): 481-485.
- ↑ 11.0 11.1 Leo, R.F. & Barghoon, E.S. 1976. Silification of wood. Bot. Mus. Leaflets Harv. Univ.' 25: 1-47.
- ↑ Seilacher, A., Reif, W.-E. & Westphal, F. 1985. Sedimentological ecological and temporal patterns of fossil Lagerstätten. In: H.B. Whittington and S. Conway Morris (eds.) Extraordinary fossil biota: their ecological and evolutionary significance. Philosophical Transactions of the Royal Society of London (B) 311: 5-23.
- ↑ 13.0 13.1 Stewart, J.M. 1977. Frozen mammoths from Siberia bring the Ice Ages to vivid life. Smithsonian Magazine, Dec.: 60-69.
- ↑ 14.0 14.1 Stankiewicz, B.A., Poinar, H.N., Briggs, D.E.G., Evershed, R.P. & Poinar, G.O.1998. Chemical Preservation of Plants and Insects in Natural Resins. Proceedings: Biological Sciences 265(1397): 641-647.
- ↑ Grimaldi, D. 1996. Amber: Window to the Past. New York: Abrams.
- ↑ 16.0 16.1 Franzen, J.L. 1985. Exceptional Preservation of Eocene Vertebrates in the Lake Deposit of Grube Messel (West Germany). Philosophical Transactions of the Royal Society of London. Series B, Biological Sciences 311(1148): 181-186.
- ↑ 17.0 17.1 Trewin, N.H. 1989. The Rhynie hot-spring deposit. Earth Science Conservation 26: 10-12.
- ↑ Gould, S.J., 1990, Wonderful life: The Burgess Shale and the Nature of History. W.W. Norton, 352 pp., ISBN 039330700X