Plant breeding: Difference between revisions
imported>David Tribe No edit summary |
Pat Palmer (talk | contribs) (→Concerns: resolving ref error) |
||
(140 intermediate revisions by 11 users not shown) | |||
Line 1: | Line 1: | ||
{{subpages}} | |||
[[Image:WheatyieldsFAOdata.jpg|frame|FAOSTAT data December 2006]] | [[Image:WheatyieldsFAOdata.jpg|frame|FAOSTAT data December 2006]] | ||
'''Plant breeding''' is the purposeful manipulation of plant species in order to create desired [[genotypes]] and [[phenotypes]] for particular purposes, such as food production, [[forestry]], and [[horticulture]]. Breeders have tried to introduce, into crop plants, traits that will increase the quality and yield of the crop; enhance their ability to tolerate harsh environmental conditions including salinity, high temperatures, and water shortage; enhance their ability to resist infection by viruses, fungi and bacteria, and enhance their ability to withstand insect pests and to tolerate herbicides. | |||
Plant breeding is an artificial version of natural [[evolution]], involving artificial selection of desired plant characteristics and artificial generation of [[genetic variation]]; it complements other farming innovations (such as introduction of new crops, [[grafting]], changed [[crop rotation]]s and tillage practices, [[irrigation]], and [[integrated pest management]]) for improving crop productivity and land stewardship. Since 1930, crop breeding, in concert with these other innovations, has led to spectacular increases in crop yields especially with cereal grains. Plant breeding has been practiced since the beginning of human civilization, but rapid advances since 1900 have been driven by concepts and techniques developed in the science of [[genetics]]. This scientific plant breeding has contributed about 50% of the improvement in crop yield seen in the major cereal crops since 1930 <ref name="Day2006">Day-Rubenstein K, Heisey P (2006) Chapter 3.1 Crop Genetic Resources, in Keith Wiebe and Noel Gollehon (Eds)(2006) ''Agricultural Resources and Environmental Indicators'' Economic Information Bulletin No. (EIB-16)</ref>. | |||
Plant breeding is | Plant breeding is practised by both [[government]] institutions and commercial enterprises. International development agencies believe that breeding new crops is important for ensuring [[food security]] and for developing [[sustainable agriculture]] by developing crops that minimize agriculture's impact on the environment<ref>[http://www.nepad.org/2005/files/documents/123.pdf Ngambeki DS (2005)] Science and technology platform for African Development: towards a green revolution in Africa, The New Partnership for Africa's Development</ref><ref>[http://www.worldbank.org/html/cgiar/publications/gef/CGIARGEF2002final.pdf Consultative Group on International Agricultural Research (2002)] Agriculture and the environment, partnership for a sustainable future</ref>. | ||
==Domestication== | |||
:''See main article [[Crop origins and evolution]]'' | |||
[[Domestication]] of plants is an [[artificial selection]] process conducted by farmers to produce plants with fewer undesirable traits than wild plants, and which makes them dependent on artificial (usually enhanced) environments for their continued existence. Many crops in present-day cultivation are the result of domestication in ancient times, about 5,000 years ago in the [[Old World]] and 3,000 years ago in the [[New World]]. In the [[Neolithic]] period, domestication took at least 1,000 years and possibly as much as 7,000 years. Today, all of our principal food crops come from domesticated varieties. | |||
[[Image:Rice_indica_japonicaPLoSs.jpg|left|frame|A bowl of indica (white, long grains) and japonica (brown, short grains) rice. [http://biology.plosjournals.org/perlserv/?request=get-document&doi=10.1371/journal.pbio.0030047 From: 'Rice genome approaches completion'] ''PLoS Biol'' 3 e47]] | |||
A cultivated crop [[species]] that has evolved from wild populations due to selective pressures from traditional [[farmer]]s is called a [[landrace]]. Landraces, which can be the result of natural forces or domestication, are plants (or animals) that are ideally suited to a particular region or environment. An example are the landraces of [[rice]], ''Oryza sativa'' [[subspecies]] ''indica'', which was developed in [[South Asia]], and ''Oryza sativa'' subspecies ''japonica'', which was developed in China. | |||
==Genetics and crop breeding intertwined== | |||
Plant breeders created the earliest artificial hybrids between different species (such as the feed cereal [[Triticale]]) in the 19th century, and progress in the science of [[genetics]] has been intertwined with crop breeding ever since. In 1903, W. L. Johannsen defined the heritable component of biological variability in experiments with a self-pollinated bean. In 1908, US plant breeder G. H. Scull discovered substantial crop yield improvements in interspecies hybrids of maize (now called 'hybrid vigor' or [[heterosis]]) with dramatic consequences for the development of a successful commercial corn-seed breeding industry, first in the USA and later elsewhere. Plant geneticists subsequently realized that hybridization can allow fuller exploitation of genetic diversity available in a plant population, and developed widely used interspecies hybrids for [[pearl millet]], [[sorghum]], [[rice]] and [[rapeseed]] (canola) crops. | |||
[[Barbara McClintock]] developed fundamental concepts about [[chromosome]] behavior and [[cytogenetics]] with [[maize]] in the 1930s. Chromosome and genome relationships both within and between crops species have been the conceptual keystones to much successful crop breeding <ref name=Jauhar>Jauhar PP (2006) Modern biotechnology as an integral supplement to conventional plant breeding: the prospects and challenges ''Crop Sci'' 46:1841-59</ref>. It is now realized that most crops have undergone duplication of the minimal [[diploid]] set of chromosome at some stage, either as ancient duplications (as in the case of [[maize]]), or by hybridization between different species (as in [[allopolyploid|allopolyploids]] such as [[wheat]]) | |||
== | Protecting major crops against disease has rested on alien gene transfer into the crop variety using chromosomal engineering. This technique relies on manipulation of chromosome pairing at [[meiosis]]. In 1956, chromosomal engineering enabled Ernest Sears to translocate a segment of goatgrass (''Agilops umbellulata'' Zhuk) chromosome that conferred resistance to [[leaf rust]] onto wheat chromosome 6B <ref>Sears ER (1956) The transfer of leaf-rust resistance from ''Aegilops umbellulata'' to wheat ''Brookhaven Symp Biol'' 9:1-22</ref>, and this achievement was followed by numerous successes in transfers of alien genes to crop plants by chromosome manipulation (the so-called wide-crosses; <ref name=Sharma>Sharma HC, Gill BS (1983) Current status of wide hybridization in wheat ''Euphytica'' 32:17-31</ref> ''see also [[wheat]]''.) | ||
The starting | |||
Chromosomal engineering is facilitated by using X-ray radiation to promote chromosome breakage, plant tissue culture, and mutants such as ''Ph-1'' of wheat that relax normal strict pairing of homologous chromosomes in meiosis <ref name=Jauhar/> <ref name=Chapman> Chapman V, Riley R (1970) Homoeologous meiotic chromosome pairing in ''Triticum aestivum'' in which chromosome 5B is replaced by an alien homoeologue ''Nature'' 226:376-7</ref> <ref name=Riley>Riley R ''et al.'' (1959) Genetic control of chromosome pairing in intergeneric hybrids with wheat ''Nature'' 183:1244-6</ref>. It can be valuable to artificially promote doubling of chromosome number using [[colchicine]] in inter-species and inter-generic cross hybrid lines as a step to enable transfer of alien chromosome segments into crop germplasm <ref>Jiang ''et al.'' (1994) Recent advances in alien gene transfer in wheat ''Euphytica'' 73:199-21</ref>. | |||
The expansion of biotechnology after 1980 led to many advances in genetics which are accelerating the usually slow process of plant breeding and leading to important discoveries that improve plant breeding, such as [[molecular marker]]s, plant [[genomics]], [[RNA interference]], and numerous gene characterizations such as the genes affecting [[abiotic stress]] responses and pest and pathogen tolerance. | |||
==Strategies for scientific plant breeding== | |||
The starting point for plant breeding is the identification of novel, useful traits in [[population]]s of parental organisms. The use of chemically or radiation induced [[mutation]] can also exploited to increase the range of useful genetic variability, and plant breeding exploits [[genetic recombination]] to generate novel combinations of traits. The end-result of lengthy selective breeding programs using these methods is "elite" varieties of high performing [[germplasm]] for major crops that are used widely in broad-acre farming. | |||
Plant breeding strategies used by the International Maize and Wheat Improvement Center (CIMMYT) encapulate the way in which modern plant breeding should be pursued. CIMMYT wheat breeding strategies aim to achieve an optimal combination of the best genotypes, in the right environments, under appropriate crop management, and appropriate to the needs of the people who must implement and manage them.<ref>[http://www.cimmyt.org/whatiscimmyt/pdf/New%20Wheats.pdf Reeves TG ''et al.'' (1999) New Wheats for a Secure, Sustainable Future. Mexico, D.F.: CIMMYT.]</ref> | |||
===Useful traits=== | ===Useful traits=== | ||
Techniques for increasing the available heritable variation in the initial population include introduction of new germplasm from distant geographical regions or from seed-bank collections, cross-pollination, either within the species, or between related species and genera - including wide-crosses using wild relatives of domesticated plants to introduce pest resistant traits needed in domesticated varieties, and creation of [[mutation]]s by irradiation or chemical treatment. | Techniques for increasing the available heritable variation in the initial population include introduction of new germplasm from distant geographical regions or from seed-bank collections, cross-pollination, either within the species, or between related species and genera - including wide-crosses using wild relatives of domesticated plants to introduce pest resistant traits needed in domesticated varieties, and creation of [[mutation]]s by irradiation or chemical treatment. | ||
===='Hope'==== | |||
In 1930, resistance to stem rust was transferred from ''T. turgidum''into wheat by producing the variety 'Hope' <ref>McFadden (1930)</ref>. In 1979, it was determined that stem rust resistance in 'Hope' was largely controlled by a single gene on the short arm of chromosome 3B. <ref>Hare and McIntosh (1979)</ref>This gene was named ''Sr2'' and assumed to have come from ''T. turgidum''. 'Hope' was used in Mexico in the 1940s as the donor for developing the stem rust resistant wheat cultivar 'Yaqui 48' <ref>(Borlaug, 1968)</ref>. Since then, the Sr2 gene has been employed widely in wheat improvement programs and distributed it to many wheat production regions of the world. The gene confers durable, broad-spectrum rust resistance effective against all isolates of ''P. graminis'', and has been described as one of the most important disease resistance genes deployed in modern plant breeding <ref>[http://intl-crop.scijournals.org/cgi/content/full/43/1/333 Spielmeyer W ''et al.'' (2003)] Identification and validation of markers linked to broad-spectrum Stem rust resistance gene Sr2 in wheat (Triticum aestivum L.) ''Crop Sci'' 43:333-6</ref>. | |||
The ''dwarfing trait'' confers a change in plant stature that provides resistance to lodging and nitrogen fertilizer responsiveness, and exploitation of this trait in wheat and rice was responsible for many of the successes of the [[Green Revolution]], which revolutionized wheat and rice production in Asia, averting mass-famine. | |||
In 1963, scientists discovered the gene ''opaque-2'', which improves the nutritional quality of maize by increasing its [[lysine]] and [[tryptophan]] content. At first, farmers initially showed little interest in opaque-2 maize because of its low yields, chalky-looking grain, and other defects. But thirty years of breeding effort finally led to releases of Quality Protein Maize (QPM) varieties, which are now widely used in regions where maize is a staple food <ref>http://www.cimmyt.org/english/wps/news/2006/aug/qpm-embu.htm The maize with the beans inside: QPM gathers a following in Kenya</ref> <ref>[http://www.cimmyt.cgiar.org/research/maize/world_food_prize_qpm/qpm_wfp.htm Scientists Receive World Food Prize for Decades-Long Scientific Quest To Produce "Quality Protein Maize" for Developing Countries]</ref>. | |||
In | |||
[[ | [[Fusarium head blight]] is a devastating disease of [[durum wheat]], and a resistant gene to fungus that causes head blight has been transferred into durum wheat germplasm from wheatgrass ''Lophopyrum elongatum'' by chromosome engineering <ref>Jauhar PP, Peterson TS (2000) Toward transferring scab resistance from a diploid wild grass, ''Lophopyrum elongatum'', into durum wheat ''Proc 2000 National Fusarium Head Blight Forum'' Cincinnati, pp 201-4</ref> | ||
===Hybrids and hybrid vigor=== | |||
Male cytoplasmic sterility and other approaches have been exploited to create artificial hybrids, and the greater vigor from [[hybrid]] vigor ([[heterosis]]) is taken advantage of in several important crops (e.g. maize, rice, canola, sorghum), whether it is a natural hybrid system of a synthetic one. | |||
[[Image:Sorghum_hybridPLoSs.jpg|frame|A field of hybrid sorghum. From: [http://biology.plosjournals.org/perlserv/?request=get-document&doi=10.1371/journal.pbio.0030039 Separating wheat from chaff in plant genomes] ''PLoS Biol'' 3:, e39]] | |||
[[ | Hybrid vigor is related partly to the presence of [[heterozygosity]] (i.e. two alternative version of the same gene). Another factor having a similar effect is [[polyploidy]], or the presence of multiple sets of chromosomes. All crop genomes are polyploid, wheat, for instance is hexaploid, and [[maize]] an ancient [[tetraploid]]. These genetic features of crop plants add extra subtlety to crop breeding and ensure that germplasm collections have great practical value <ref>Udall JA, Wendel JF (2006) Polyploidy and crop improvement ''Crop Sci'' 46:S-3-S-14 </ref> <ref>Odland W ''et al.'' (2006) Ancestral rice blocks Define multiple related regions in the maize genome ''Crop Sci'' 46:41-8</ref> . | ||
===Recombination=== | |||
As well as taking advantage of natural [[gene flow]] and [[horizontal gene transfer]] events to allow new traits to be added to existing crops, it also uses artificial means for gene transfer such as embryo rescue and biolistics to overcome natural barriers to [[gene flow]] between different gene-pools. | |||
[[Genetic engineering]] to generate [[transgenic plant]]s, and gene silencing (called [[RNA interference]] or cisgenics) are other methods used to obtain useful variants. | |||
[[ | Barriers to gene-flow between different plant species are overcome in a number of ways. [[Colchicine]] treatment to create artificial [[polyploid]]s can overcome some of the sterility problems from inter-species cross-pollination. Cochicine interferes with meiosis, and results in formation of diploid rather than haploid [[gametes]]. Crosses made using protoplast fusion ([[somatic hybridization]]) can also be used, and embryo rescue methods can also circumvent gene-flow barriers. | ||
===Improved selection of better varieties=== | |||
Many [[artificial selection]] methods have been developed for crop improvement. These include [[molecular marker]] assisted breeding<ref>[http://maswheat.ucdavis.edu/ Coordinated Agricultural project UC Davis]</ref> <ref>Bilyeu K ''et al.'' (2006) Molecular genetic resources for development of 1% linolenic acid soybeans ''Crop Sci'' 46:1913-8 DOI: 10.2135/cropsci2005.11-0426</ref>, and use of statistical principles to design field tests of crop candidate performance. | |||
[[Genome]] science (chromosome sequence decoding and computer-assisted dissection of gene functions and structure) is also being used to help identify traits and select improved progeny. One approach is to compare genes in different species (comparative genomics) to take advantage of the greater ease of gene sequencing with small, compact genomes such as those of ''[[Arabidopsis thaliana]]'', or of rice. Of the main cereal crops, rice has the smallest genome; at least three rice genomes have been analyzed in terms of detailed gene sequence, already yielding much information about the repeated genome, chromosome and gene duplications that have occurred in this species during natural evolution <ref>[http://biology.plosjournals.org/perlserv/?request=get-document&doi=10.1371/journal.pbio.0030047 Rice Genome Approaches Completion] ''PLoS Biol'' 3: e47 doi:10.1371/journal.pbio.0030047</ref><ref>[http://biology.plosjournals.org/perlserv/?request=get-document&doi=10.1371/journal.pbio.0030038 Yu J ''et al.'' (2005]) The genomes of Oryza sativa: a history of duplications ''PLoS Biol'' 3: e38 doi:10.1371/journal.pbio.0030038</ref>. | |||
Identifying the particular [[gene]] and [[DNA sequence]]s that determine a [[phenotype]] of relevance to agriculture (such as resistance to rust fungal pathogens) opens up many ways of creating new, useful genetic variation by direct manipulation of DNA, and for devising tests for tracking traits such as water use efficiency <ref>[http://www.csiro.au/files/mediaRelease/mr2004/Prdrysdale2.htm CSIRO Media Release - Ref 2004/33 - Mar 04 , 2004 Huge potential for water-efficient wheat (Delta carbon technology).]</ref> and pathogen resistance which are difficult to identify in breeding experiments, thus speeding up plant breeding in the greenhouse and field trial stages. For instance, [[microsatellite]] molecular markers have been developed that are useful for detecting the important Stem rust resistance trait (Sr2, present in cultivar 'Hope') in wheat. <ref>[http://intl-crop.scijournals.org/cgi/content/full/43/1/333Spielmeyer W ''et al.'' (2003)] Identification and validation of markers linked to broad-spectrum stem rust resistance gene Sr2 in wheat (Triticum aestivum L.) ''Crop Sci'' 43:333-6</ref>. | |||
==Germplasm collections== | |||
In the 1930s, Russian scientist [[Nikolai Vavilov]] called attention to the value of wild crop relatives as a source of genes for improving agriculture, and in travels over five continents he amassed the largest collection of (at that time) of species and strains of cultivated plants in the world. <ref>[Tanksley SD, McCouch SR (1997) Seed banks and molecular maps: unlocking genetic potential from the wild ''Science'' 277(5329):1063-6. citing N. I. Vavilov, in The New Systematics, J. Huxley, Ed. (Clarendon, Oxford, 1940), pp. 549–566.]</ref> <ref>[http://www.ifpri.org/pubs/otherpubs/savingseeds.htm B Koo, International Food Policy Research Institute, (IFPRI), Washington D C, USA; P G Pardey, University of Minnesota, USA; B D Wright, University of California, Berkeley, USA, and others. (2004). Saving Seeds: The Economics of Conserving Crop Genetic Resources ex situ in the future harvest centres of CGIAR, page 1 citing Resnick S and Vavilov Y (1997) The Russian Scientist Nicolay Vavilov. Preface to the English translation of Five Continents by N. I Vavilov, International Plant Genetic Resources Institute, Rome.]</ref> His intent was to promote crop improvement, but since then, other considerations have added to expansion of seed-banks. One major concern is the limited genetic diversity of crop plants, and the vulnerabilities to crop diseases that it introduces into the food supply. This was highlighted in 1970 by a severe outbreak of Southern corn leaf blight in the USA. | |||
CGIAR | The Food and Agriculture Organization (FAO) of the United Nations estimate that globally, distinct seed samples in plant seed collections total over 6 million samples, held in in 1300 genebanks worldwide (B. Koo ''et al.'' in ''Saving Seeds'' 2004, citing FAO 1998). About 10% of these are held by the substantial international network of crop germplasm collections managed by the Consultative Group on International Agricultural Research (CGIAR) <ref>[http://www.cgiar.org/who/index.html Consultative Group on International Agricultural Research (CGIAR)].</ref> (CGIAR was created in 1971 as a strategic alliance of countries, international and regional organizations, and private foundations supporting 15 international agricultural centers). CGIAR genbanks include | ||
* [http://www.cimmyt.org/ Centro Internacional de Mejoramiento de Maiz y Trigo (maize and wheat; CIMMYT) Genebank], (Mexico. | * [http://www.cimmyt.org/ Centro Internacional de Mejoramiento de Maiz y Trigo (maize and wheat; CIMMYT) Genebank], (Mexico. | ||
* [http://www.icarda.cgiar.org/ International Center for Agricultural Research in the Dry Areas (ICARDA) Genebank], (Syria). | * [http://www.icarda.cgiar.org/ International Center for Agricultural Research in the Dry Areas (ICARDA) Genebank], (Syria). | ||
* [http://www.icrisat.org/ International Crops Research Institute for the Semi-Arid Tropics (ICRISAT) Genebank], (India). | * [http://www.icrisat.org/ International Crops Research Institute for the Semi-Arid Tropics (ICRISAT) Genebank], (India). | ||
* [http://www.irri.org/ International Rice Research Institute IRRI Genebank], ( | * [http://www.irri.org/ International Rice Research Institute IRRI Genebank], (Philippines). | ||
* [http://www.ciat.cgiar.org/Centro Internacional de Agricultura Tropical (International Center for Tropical Agriculture; CIAT) Genebank], (Columbia) | * [http://www.ciat.cgiar.org/Centro Internacional de Agricultura Tropical (International Center for Tropical Agriculture; CIAT) Genebank], (Columbia) | ||
Line 62: | Line 79: | ||
* New Rices for Africa (NERICAs) from [http://www.warda.cgiar.org/ Africa Rice Center (WARDA)] that are transforming agriculture in the West Africa region. In 2003 it is estimated that NERICAs were planted on 23,000 hectares, and their use is spreading across Africa, for instance to Uganda and Guinea. | * New Rices for Africa (NERICAs) from [http://www.warda.cgiar.org/ Africa Rice Center (WARDA)] that are transforming agriculture in the West Africa region. In 2003 it is estimated that NERICAs were planted on 23,000 hectares, and their use is spreading across Africa, for instance to Uganda and Guinea. | ||
* Release across Latin America of many new bean varieties and improved forages that are grown on over 100 million hectares in that region. | * Release across Latin America of many new bean varieties and improved forages that are grown on over 100 million hectares in that region. | ||
==Concerns== | |||
Modern plant breeding, whether classical or through genetic engineering, raises issues of concern, particularly with regard to food crops. Surveys of changes in American foods 1950-1999 have suggested there may be decreases in nutitional quality of many garden crops over this period, possibly because of breeding for higher yield. <ref>[http://www.jacn.org/cgi/content/abstract/23/6/669?maxtoshow=&HITS=10&hits=10&RESULTFORMAT=&author1=donald+davis&andorexacttitle=and&andorexacttitleabs=and&andorexactfulltext=and&searchid=1110477116345_697&stored_search=&FIRSTINDEX=0&sortspec=relevance&journalcode=jamcnutr Davis, DR ''et al.'' (2004) Changes in USDA Food Composition Data for 43 Garden Crops 1950 to 1999. ''J Am College Nutrition'' 23:669-82]</ref> | |||
Such problems are not new. At the beginning of agriculture, a gene was lost from wheat that mobilizes nutrients from leaves, causing better grain yields at the expense of protein content and nutritional value <ref>[http://www.eurekalert.org/pub_releases/2006-11/uoc--wgm111706.php Wheat gene may boost foods' nutrient content]</ref> <ref>Uauy C ''et al.'' (2006) A NAC Gene Regulating Senescence Improves grain protein, zinc, and iron Content in Wheat ''Science'' 314:1298-301 | |||
:'' | DOI: 10.1126/science.1133649</ref>. Among the many varieties of wheat used in modern times, there is an inverse relationship between yield and protein content.<ref>Simmonds NW (1995) The relation between yield and protein in cereal grain ''J Sci Food Agric'' 67:309-15</ref>. There is also increasing recent emphasis on breeding crops for nutritional improvement<ref>[http://www.harvestplus.org/ HarvestPlus, an international, interdisciplinary, research program that seeks to reduce micronutrient malnutrition by harnessing the powers of agriculture and nutrition research to breed nutrient dense staple foods.]</ref>. | ||
:'' | |||
: | |||
There is considerable debate about genetic modification of plants, encompassing the [[Ecological impact of transgenic plants|ecological impact of genetically modified plants]] and the safety of [[genetically modified food]]. It extends also to the issue of [[Food security]] because of the link between increases in crop output and matching of food supply to growing food demand caused by population growth and economic growth. Agencies such as the International Food Policy Research Institute (IFPRI) have highlighted the mis-match between amount of agricultural R&D and food security in the developing world <ref>[http://www.ifpri.org/pubs/books/oc51.asp Pardey PG ''et al.'' eds. (2006) Agricultural R&D in the Developing World Too Little, Too Late]</ref>. | |||
[[Plant breeders' rights]] is also a controversial issue. Efforts to strengthen breeders' rights, for example, by lengthening periods of variety protection, are ongoing. Today, production of new varieties is dominated by commercial plant breeders, who seek to protect their work and collect royalties through national and international agreements based in [[intellectual property rights]]. | |||
Put simply, critics of crop-breeding argue that, through a combination of technical and economic pressures, commercial breeders are reducing [[biodiversity]] and significantly constraining farmers from developing and trading seed on a regional level. | |||
But seed breeding is a specialized economic activity that most farmers do not have the time to pursue, and better seed provides a simple means of technology transfer that provides an economic benefit to the farmer. Expansion of a commercialized seed industry is historically associated with substantial economic gains in that sector as illustrated by hybrid maize in the USA, and more recently, the Indian cotton seed industry <ref>[http://www.fbae.org/News/performance_of_bt_cotton_in_indi.htm Performance of Bt cotton in India: The 2005-06 Season], Foundation for Biotechnology Awareness and Education, Bangalore, India</ref> <ref>[http://www.isid.ac.in/~planning/workingpapers/dp06-05.pdf Murugkar M ''et al.''] (2006) Liberalization, Biotechnology and the Private Seed Sector: The Case of India’s Cotton Seed Market Discussion Paper 06-05, Indian Statistical Institute, Delhi</ref> | |||
<ref>[http://www.nature.com/nrg/journal/v2/n1/abs/nrg0101_069a_fs.html;jsessionid=7AF3CABB24BC62D912EA5F052D7D5C38 Duvick DN. (2001)] Biotechnology in the 1930s: the development of hybrid maize ''Nat Rev Genet'' 2:69-74</ref>. Critics of excessive precautionary regulation argue that costly regulatory burdens and delays imposed on new seed-breeding technologies restrict investment in much modern agricultural technology to organizations having substantial financial assets, which limits the effectiveness of public research efforts in developing countries. | |||
But seed breeding is a | |||
<ref>[http://www.nature.com/nrg/journal/v2/n1/abs/nrg0101_069a_fs.html;jsessionid=7AF3CABB24BC62D912EA5F052D7D5C38 Duvick DN. (2001) Biotechnology in the 1930s: the development of hybrid maize | |||
==References== | ==References== | ||
= | <div class="references-small" style="-moz-column-count:2; column-count:2;"> | ||
<references /> | |||
</div> | |||
Revision as of 08:09, 1 August 2024

Plant breeding is the purposeful manipulation of plant species in order to create desired genotypes and phenotypes for particular purposes, such as food production, forestry, and horticulture. Breeders have tried to introduce, into crop plants, traits that will increase the quality and yield of the crop; enhance their ability to tolerate harsh environmental conditions including salinity, high temperatures, and water shortage; enhance their ability to resist infection by viruses, fungi and bacteria, and enhance their ability to withstand insect pests and to tolerate herbicides.
Plant breeding is an artificial version of natural evolution, involving artificial selection of desired plant characteristics and artificial generation of genetic variation; it complements other farming innovations (such as introduction of new crops, grafting, changed crop rotations and tillage practices, irrigation, and integrated pest management) for improving crop productivity and land stewardship. Since 1930, crop breeding, in concert with these other innovations, has led to spectacular increases in crop yields especially with cereal grains. Plant breeding has been practiced since the beginning of human civilization, but rapid advances since 1900 have been driven by concepts and techniques developed in the science of genetics. This scientific plant breeding has contributed about 50% of the improvement in crop yield seen in the major cereal crops since 1930 [1].
Plant breeding is practised by both government institutions and commercial enterprises. International development agencies believe that breeding new crops is important for ensuring food security and for developing sustainable agriculture by developing crops that minimize agriculture's impact on the environment[2][3].
Domestication
- See main article Crop origins and evolution
Domestication of plants is an artificial selection process conducted by farmers to produce plants with fewer undesirable traits than wild plants, and which makes them dependent on artificial (usually enhanced) environments for their continued existence. Many crops in present-day cultivation are the result of domestication in ancient times, about 5,000 years ago in the Old World and 3,000 years ago in the New World. In the Neolithic period, domestication took at least 1,000 years and possibly as much as 7,000 years. Today, all of our principal food crops come from domesticated varieties.
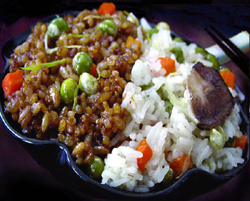
A cultivated crop species that has evolved from wild populations due to selective pressures from traditional farmers is called a landrace. Landraces, which can be the result of natural forces or domestication, are plants (or animals) that are ideally suited to a particular region or environment. An example are the landraces of rice, Oryza sativa subspecies indica, which was developed in South Asia, and Oryza sativa subspecies japonica, which was developed in China.
Genetics and crop breeding intertwined
Plant breeders created the earliest artificial hybrids between different species (such as the feed cereal Triticale) in the 19th century, and progress in the science of genetics has been intertwined with crop breeding ever since. In 1903, W. L. Johannsen defined the heritable component of biological variability in experiments with a self-pollinated bean. In 1908, US plant breeder G. H. Scull discovered substantial crop yield improvements in interspecies hybrids of maize (now called 'hybrid vigor' or heterosis) with dramatic consequences for the development of a successful commercial corn-seed breeding industry, first in the USA and later elsewhere. Plant geneticists subsequently realized that hybridization can allow fuller exploitation of genetic diversity available in a plant population, and developed widely used interspecies hybrids for pearl millet, sorghum, rice and rapeseed (canola) crops.
Barbara McClintock developed fundamental concepts about chromosome behavior and cytogenetics with maize in the 1930s. Chromosome and genome relationships both within and between crops species have been the conceptual keystones to much successful crop breeding [4]. It is now realized that most crops have undergone duplication of the minimal diploid set of chromosome at some stage, either as ancient duplications (as in the case of maize), or by hybridization between different species (as in allopolyploids such as wheat)
Protecting major crops against disease has rested on alien gene transfer into the crop variety using chromosomal engineering. This technique relies on manipulation of chromosome pairing at meiosis. In 1956, chromosomal engineering enabled Ernest Sears to translocate a segment of goatgrass (Agilops umbellulata Zhuk) chromosome that conferred resistance to leaf rust onto wheat chromosome 6B [5], and this achievement was followed by numerous successes in transfers of alien genes to crop plants by chromosome manipulation (the so-called wide-crosses; [6] see also wheat.)
Chromosomal engineering is facilitated by using X-ray radiation to promote chromosome breakage, plant tissue culture, and mutants such as Ph-1 of wheat that relax normal strict pairing of homologous chromosomes in meiosis [4] [7] [8]. It can be valuable to artificially promote doubling of chromosome number using colchicine in inter-species and inter-generic cross hybrid lines as a step to enable transfer of alien chromosome segments into crop germplasm [9].
The expansion of biotechnology after 1980 led to many advances in genetics which are accelerating the usually slow process of plant breeding and leading to important discoveries that improve plant breeding, such as molecular markers, plant genomics, RNA interference, and numerous gene characterizations such as the genes affecting abiotic stress responses and pest and pathogen tolerance.
Strategies for scientific plant breeding
The starting point for plant breeding is the identification of novel, useful traits in populations of parental organisms. The use of chemically or radiation induced mutation can also exploited to increase the range of useful genetic variability, and plant breeding exploits genetic recombination to generate novel combinations of traits. The end-result of lengthy selective breeding programs using these methods is "elite" varieties of high performing germplasm for major crops that are used widely in broad-acre farming.
Plant breeding strategies used by the International Maize and Wheat Improvement Center (CIMMYT) encapulate the way in which modern plant breeding should be pursued. CIMMYT wheat breeding strategies aim to achieve an optimal combination of the best genotypes, in the right environments, under appropriate crop management, and appropriate to the needs of the people who must implement and manage them.[10]
Useful traits
Techniques for increasing the available heritable variation in the initial population include introduction of new germplasm from distant geographical regions or from seed-bank collections, cross-pollination, either within the species, or between related species and genera - including wide-crosses using wild relatives of domesticated plants to introduce pest resistant traits needed in domesticated varieties, and creation of mutations by irradiation or chemical treatment.
'Hope'
In 1930, resistance to stem rust was transferred from T. turgiduminto wheat by producing the variety 'Hope' [11]. In 1979, it was determined that stem rust resistance in 'Hope' was largely controlled by a single gene on the short arm of chromosome 3B. [12]This gene was named Sr2 and assumed to have come from T. turgidum. 'Hope' was used in Mexico in the 1940s as the donor for developing the stem rust resistant wheat cultivar 'Yaqui 48' [13]. Since then, the Sr2 gene has been employed widely in wheat improvement programs and distributed it to many wheat production regions of the world. The gene confers durable, broad-spectrum rust resistance effective against all isolates of P. graminis, and has been described as one of the most important disease resistance genes deployed in modern plant breeding [14].
The dwarfing trait confers a change in plant stature that provides resistance to lodging and nitrogen fertilizer responsiveness, and exploitation of this trait in wheat and rice was responsible for many of the successes of the Green Revolution, which revolutionized wheat and rice production in Asia, averting mass-famine.
In 1963, scientists discovered the gene opaque-2, which improves the nutritional quality of maize by increasing its lysine and tryptophan content. At first, farmers initially showed little interest in opaque-2 maize because of its low yields, chalky-looking grain, and other defects. But thirty years of breeding effort finally led to releases of Quality Protein Maize (QPM) varieties, which are now widely used in regions where maize is a staple food [15] [16].
Fusarium head blight is a devastating disease of durum wheat, and a resistant gene to fungus that causes head blight has been transferred into durum wheat germplasm from wheatgrass Lophopyrum elongatum by chromosome engineering [17]
Hybrids and hybrid vigor
Male cytoplasmic sterility and other approaches have been exploited to create artificial hybrids, and the greater vigor from hybrid vigor (heterosis) is taken advantage of in several important crops (e.g. maize, rice, canola, sorghum), whether it is a natural hybrid system of a synthetic one.
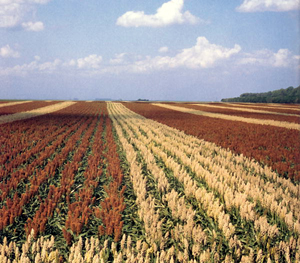
Hybrid vigor is related partly to the presence of heterozygosity (i.e. two alternative version of the same gene). Another factor having a similar effect is polyploidy, or the presence of multiple sets of chromosomes. All crop genomes are polyploid, wheat, for instance is hexaploid, and maize an ancient tetraploid. These genetic features of crop plants add extra subtlety to crop breeding and ensure that germplasm collections have great practical value [18] [19] .
Recombination
As well as taking advantage of natural gene flow and horizontal gene transfer events to allow new traits to be added to existing crops, it also uses artificial means for gene transfer such as embryo rescue and biolistics to overcome natural barriers to gene flow between different gene-pools.
Genetic engineering to generate transgenic plants, and gene silencing (called RNA interference or cisgenics) are other methods used to obtain useful variants.
Barriers to gene-flow between different plant species are overcome in a number of ways. Colchicine treatment to create artificial polyploids can overcome some of the sterility problems from inter-species cross-pollination. Cochicine interferes with meiosis, and results in formation of diploid rather than haploid gametes. Crosses made using protoplast fusion (somatic hybridization) can also be used, and embryo rescue methods can also circumvent gene-flow barriers.
Improved selection of better varieties
Many artificial selection methods have been developed for crop improvement. These include molecular marker assisted breeding[20] [21], and use of statistical principles to design field tests of crop candidate performance.
Genome science (chromosome sequence decoding and computer-assisted dissection of gene functions and structure) is also being used to help identify traits and select improved progeny. One approach is to compare genes in different species (comparative genomics) to take advantage of the greater ease of gene sequencing with small, compact genomes such as those of Arabidopsis thaliana, or of rice. Of the main cereal crops, rice has the smallest genome; at least three rice genomes have been analyzed in terms of detailed gene sequence, already yielding much information about the repeated genome, chromosome and gene duplications that have occurred in this species during natural evolution [22][23].
Identifying the particular gene and DNA sequences that determine a phenotype of relevance to agriculture (such as resistance to rust fungal pathogens) opens up many ways of creating new, useful genetic variation by direct manipulation of DNA, and for devising tests for tracking traits such as water use efficiency [24] and pathogen resistance which are difficult to identify in breeding experiments, thus speeding up plant breeding in the greenhouse and field trial stages. For instance, microsatellite molecular markers have been developed that are useful for detecting the important Stem rust resistance trait (Sr2, present in cultivar 'Hope') in wheat. [25].
Germplasm collections
In the 1930s, Russian scientist Nikolai Vavilov called attention to the value of wild crop relatives as a source of genes for improving agriculture, and in travels over five continents he amassed the largest collection of (at that time) of species and strains of cultivated plants in the world. [26] [27] His intent was to promote crop improvement, but since then, other considerations have added to expansion of seed-banks. One major concern is the limited genetic diversity of crop plants, and the vulnerabilities to crop diseases that it introduces into the food supply. This was highlighted in 1970 by a severe outbreak of Southern corn leaf blight in the USA.
The Food and Agriculture Organization (FAO) of the United Nations estimate that globally, distinct seed samples in plant seed collections total over 6 million samples, held in in 1300 genebanks worldwide (B. Koo et al. in Saving Seeds 2004, citing FAO 1998). About 10% of these are held by the substantial international network of crop germplasm collections managed by the Consultative Group on International Agricultural Research (CGIAR) [28] (CGIAR was created in 1971 as a strategic alliance of countries, international and regional organizations, and private foundations supporting 15 international agricultural centers). CGIAR genbanks include
- Centro Internacional de Mejoramiento de Maiz y Trigo (maize and wheat; CIMMYT) Genebank, (Mexico.
- International Center for Agricultural Research in the Dry Areas (ICARDA) Genebank, (Syria).
- International Crops Research Institute for the Semi-Arid Tropics (ICRISAT) Genebank, (India).
- International Rice Research Institute IRRI Genebank, (Philippines).
- Internacional de Agricultura Tropical (International Center for Tropical Agriculture; CIAT) Genebank, (Columbia)
Recent achievements coming from this germplasm resource and the associated CGIAR network of scientists include:
- Quality Protein Maize (QPM) varieties have been released in 25 countries, and are grown on more than 600,000 hectares
- New Rices for Africa (NERICAs) from Africa Rice Center (WARDA) that are transforming agriculture in the West Africa region. In 2003 it is estimated that NERICAs were planted on 23,000 hectares, and their use is spreading across Africa, for instance to Uganda and Guinea.
- Release across Latin America of many new bean varieties and improved forages that are grown on over 100 million hectares in that region.
Concerns
Modern plant breeding, whether classical or through genetic engineering, raises issues of concern, particularly with regard to food crops. Surveys of changes in American foods 1950-1999 have suggested there may be decreases in nutitional quality of many garden crops over this period, possibly because of breeding for higher yield. [29]
Such problems are not new. At the beginning of agriculture, a gene was lost from wheat that mobilizes nutrients from leaves, causing better grain yields at the expense of protein content and nutritional value [30] [31]. Among the many varieties of wheat used in modern times, there is an inverse relationship between yield and protein content.[32]. There is also increasing recent emphasis on breeding crops for nutritional improvement[33].
There is considerable debate about genetic modification of plants, encompassing the ecological impact of genetically modified plants and the safety of genetically modified food. It extends also to the issue of Food security because of the link between increases in crop output and matching of food supply to growing food demand caused by population growth and economic growth. Agencies such as the International Food Policy Research Institute (IFPRI) have highlighted the mis-match between amount of agricultural R&D and food security in the developing world [34].
Plant breeders' rights is also a controversial issue. Efforts to strengthen breeders' rights, for example, by lengthening periods of variety protection, are ongoing. Today, production of new varieties is dominated by commercial plant breeders, who seek to protect their work and collect royalties through national and international agreements based in intellectual property rights.
Put simply, critics of crop-breeding argue that, through a combination of technical and economic pressures, commercial breeders are reducing biodiversity and significantly constraining farmers from developing and trading seed on a regional level.
But seed breeding is a specialized economic activity that most farmers do not have the time to pursue, and better seed provides a simple means of technology transfer that provides an economic benefit to the farmer. Expansion of a commercialized seed industry is historically associated with substantial economic gains in that sector as illustrated by hybrid maize in the USA, and more recently, the Indian cotton seed industry [35] [36] [37]. Critics of excessive precautionary regulation argue that costly regulatory burdens and delays imposed on new seed-breeding technologies restrict investment in much modern agricultural technology to organizations having substantial financial assets, which limits the effectiveness of public research efforts in developing countries.
References
- ↑ Day-Rubenstein K, Heisey P (2006) Chapter 3.1 Crop Genetic Resources, in Keith Wiebe and Noel Gollehon (Eds)(2006) Agricultural Resources and Environmental Indicators Economic Information Bulletin No. (EIB-16)
- ↑ Ngambeki DS (2005) Science and technology platform for African Development: towards a green revolution in Africa, The New Partnership for Africa's Development
- ↑ Consultative Group on International Agricultural Research (2002) Agriculture and the environment, partnership for a sustainable future
- ↑ 4.0 4.1 Jauhar PP (2006) Modern biotechnology as an integral supplement to conventional plant breeding: the prospects and challenges Crop Sci 46:1841-59
- ↑ Sears ER (1956) The transfer of leaf-rust resistance from Aegilops umbellulata to wheat Brookhaven Symp Biol 9:1-22
- ↑ Sharma HC, Gill BS (1983) Current status of wide hybridization in wheat Euphytica 32:17-31
- ↑ Chapman V, Riley R (1970) Homoeologous meiotic chromosome pairing in Triticum aestivum in which chromosome 5B is replaced by an alien homoeologue Nature 226:376-7
- ↑ Riley R et al. (1959) Genetic control of chromosome pairing in intergeneric hybrids with wheat Nature 183:1244-6
- ↑ Jiang et al. (1994) Recent advances in alien gene transfer in wheat Euphytica 73:199-21
- ↑ Reeves TG et al. (1999) New Wheats for a Secure, Sustainable Future. Mexico, D.F.: CIMMYT.
- ↑ McFadden (1930)
- ↑ Hare and McIntosh (1979)
- ↑ (Borlaug, 1968)
- ↑ Spielmeyer W et al. (2003) Identification and validation of markers linked to broad-spectrum Stem rust resistance gene Sr2 in wheat (Triticum aestivum L.) Crop Sci 43:333-6
- ↑ http://www.cimmyt.org/english/wps/news/2006/aug/qpm-embu.htm The maize with the beans inside: QPM gathers a following in Kenya
- ↑ Scientists Receive World Food Prize for Decades-Long Scientific Quest To Produce "Quality Protein Maize" for Developing Countries
- ↑ Jauhar PP, Peterson TS (2000) Toward transferring scab resistance from a diploid wild grass, Lophopyrum elongatum, into durum wheat Proc 2000 National Fusarium Head Blight Forum Cincinnati, pp 201-4
- ↑ Udall JA, Wendel JF (2006) Polyploidy and crop improvement Crop Sci 46:S-3-S-14
- ↑ Odland W et al. (2006) Ancestral rice blocks Define multiple related regions in the maize genome Crop Sci 46:41-8
- ↑ Coordinated Agricultural project UC Davis
- ↑ Bilyeu K et al. (2006) Molecular genetic resources for development of 1% linolenic acid soybeans Crop Sci 46:1913-8 DOI: 10.2135/cropsci2005.11-0426
- ↑ Rice Genome Approaches Completion PLoS Biol 3: e47 doi:10.1371/journal.pbio.0030047
- ↑ Yu J et al. (2005) The genomes of Oryza sativa: a history of duplications PLoS Biol 3: e38 doi:10.1371/journal.pbio.0030038
- ↑ CSIRO Media Release - Ref 2004/33 - Mar 04 , 2004 Huge potential for water-efficient wheat (Delta carbon technology).
- ↑ W et al. (2003) Identification and validation of markers linked to broad-spectrum stem rust resistance gene Sr2 in wheat (Triticum aestivum L.) Crop Sci 43:333-6
- ↑ [Tanksley SD, McCouch SR (1997) Seed banks and molecular maps: unlocking genetic potential from the wild Science 277(5329):1063-6. citing N. I. Vavilov, in The New Systematics, J. Huxley, Ed. (Clarendon, Oxford, 1940), pp. 549–566.]
- ↑ B Koo, International Food Policy Research Institute, (IFPRI), Washington D C, USA; P G Pardey, University of Minnesota, USA; B D Wright, University of California, Berkeley, USA, and others. (2004). Saving Seeds: The Economics of Conserving Crop Genetic Resources ex situ in the future harvest centres of CGIAR, page 1 citing Resnick S and Vavilov Y (1997) The Russian Scientist Nicolay Vavilov. Preface to the English translation of Five Continents by N. I Vavilov, International Plant Genetic Resources Institute, Rome.
- ↑ Consultative Group on International Agricultural Research (CGIAR).
- ↑ Davis, DR et al. (2004) Changes in USDA Food Composition Data for 43 Garden Crops 1950 to 1999. J Am College Nutrition 23:669-82
- ↑ Wheat gene may boost foods' nutrient content
- ↑ Uauy C et al. (2006) A NAC Gene Regulating Senescence Improves grain protein, zinc, and iron Content in Wheat Science 314:1298-301 DOI: 10.1126/science.1133649
- ↑ Simmonds NW (1995) The relation between yield and protein in cereal grain J Sci Food Agric 67:309-15
- ↑ HarvestPlus, an international, interdisciplinary, research program that seeks to reduce micronutrient malnutrition by harnessing the powers of agriculture and nutrition research to breed nutrient dense staple foods.
- ↑ Pardey PG et al. eds. (2006) Agricultural R&D in the Developing World Too Little, Too Late
- ↑ Performance of Bt cotton in India: The 2005-06 Season, Foundation for Biotechnology Awareness and Education, Bangalore, India
- ↑ Murugkar M et al. (2006) Liberalization, Biotechnology and the Private Seed Sector: The Case of India’s Cotton Seed Market Discussion Paper 06-05, Indian Statistical Institute, Delhi
- ↑ Duvick DN. (2001) Biotechnology in the 1930s: the development of hybrid maize Nat Rev Genet 2:69-74